Posts by Align Manufacturing
Why Manufacturers Need to Get Out of China Now: Strategic Insights from Align Manufacturing
In this video, Casey Ross and Benjamin Unruh from Align Manufacturing and Alex Chiu from Maesot Heavy Industries discuss why manufacturers must urgently consider moving their operations out of China. They explore the impact of geopolitical tensions, rising tariffs, and the key strategies for ensuring a smooth transition to Southeast and South Asia.
Casey: Hi everyone, I'm Casey Ross, Partner at Align Manufacturing. I'm here today with Benjamin Unruh, Partner at Align Manufacturing, and Alex Chiu, Deputy General Manager at Maesot Heavy Industries, to discuss the critical shifts in global manufacturing landscapes and the strategic importance of relocating production from China to more favorable markets in Southeast and South Asia. Ben, Alex, thank you for joining us here on this podcast. Alex, why don't you introduce yourself? You have a very interesting background. So please, tell us about your background and also a little bit about your business.
Alex: Hi. Thank you, Casey and Ben for having me in this podcast. My name is Alex. I'm from Maesot Heavy Industries. Um, we are actually a family owned business. We predominantly do foundry and sand casting, furan resin, green sand and shell molding. I actually graduated from the US with a bachelors in mechanical engineering. We have been in Thailand for a bit more than I would say 8 to 9 years. Yeah. And, we work with many different companies, including foreign like North American companies, Japanese companies, and of course the local Thai industries. Right.
Casey: And I think you actually, you have a really interesting background because being Taiwanese and living in Thailand, owning a factory here gives you a very interesting perspective on what we were going to discuss in this podcast. So yeah, thank you for joining us. So, let's let's dive into it. What is driving the necessity for OEMs to get out of China, or at least have a China plus one?
Ben: Yeah, I mean, the the big one on everyone's mind in our industry is, of course, the tariffs, the duty, the 301 tariffs, which were started under the Trump administration and continued under Biden. It's kind of been a progressive tax on imports from China that started off small and a little bit negligible and have scaled up. And so, you know, every business, every importer is and should be concerned with their bottom line. And, you know, these punitive tariffs that were put in place by the Customs and Border Protection, they wanted to, they're enacted to encourage American businesses to divest from China and seek alternatives. So, you know, that's probably the main driver. But there are some other points as well.
Casey: Sure. And Alex, how have you seen the tariffs impact your business?
Alex: For me as a manufacturer, I would say it's a positive impact for me. We have many customers originally ordering from China due to the tariffs, the cost constraints and they are slowly starting to order from us, a non-Chinese source. So, like for business wise, it's actually a good thing for me. Yeah.
Ben: And yeah, I mean, I would just say, you know, the cost is one thing and that's, you know, first and foremost on everyone's mind. But there's other factors as well. And large companies, like Apple and Sony and multinationals all over the world that, you know, saw this and in kind of earnest and started divesting from China to alternative markets like here in Thailand or Vietnam, you know, more than a decade ago. But now it's kind of small and medium enterprises are making the switch and experiencing, you know, some growing pains associated with that. But, you know, ultimately, it's important for importers to be able to have free access to their goods, and things like increasing authoritarianism in China, clamp down on Western businesses, and just general saber rattling on the geopolitical stage all present pretty large risks to importers who are solely reliant on Chinese manufacturers for their imports.
Casey: I think you bring up a really good point because, you know, like you said, like 10, 15 years ago, these large companies like Panasonic and Samsung, I mean, they started moving their manufacturing a long time ago even before the tariffs, because, you know, I think they saw something coming. And it's true, like a lot of these SME companies, they're now making the jump and they're experiencing a lot of pains, you know, moving their supply chains. So I mean, with your business, is your factory capacity filling up quite quickly?
Alex: Yeah, we actually especially before Covid but especially after Covid, I think people seeing the situation in China. We constantly get more orders each year from like from compared to last year. We also our sales volume increased mostly from the redirected orders.
Casey: So, you know, I would like to talk about maybe the differences between the three countries that we actually work in. So we work in India, Thailand, and Vietnam. And I think, you know, every country has its competitive advantages and its noncompetitive advantages. So, maybe let's talk about the differences between these countries. Let's start off, Ben, like what do you see out of India?
Ben: Yeah, India, you know, it's a huge manufacturing market. It's probably the number two for heavy industrial outside of China. So, India is relatively new as an import source for American companies, but they've been exporting to countries in Asia, particularly in the Middle East as well as Africa, for a long time as the primary supplier. You know, India has a lot of foundries, a lot of forges, there's multiple regions that are kind of manufacturing hubs. At a really high level, you can get great stuff out of India, and it's probably the most cost competitive option of the three. Where you really have to tread carefully is choosing the correct partner. And, there's a lot of incorrect partners out there where, you know, they may promise you that everything is going to be great and you might get your sample, and it just doesn't always work out that way. And so, you know, a lot of what we do behind the scenes when we're not in a studio like this is we're in country. I've been to India four times this year. And we're driving around and we're visiting foundries. We're checking up on our existing projects and always looking for, you know, the next great supplier. And so, to try to just choose someone off of Google, it's a risky proposition. I would say you don't always have the best bet.
Casey: I would say in India, everyone says, yes, it can be done. And then after you sort of start digging down, you find out very quickly that they just say yes to everyone. Yeah. I think that's one of the biggest pitfalls that we see in India and also the infrastructure. You have to be very careful about picking a partner, not just for them, but also their location. You know, I'll give you just a quick example. We were auditing. We were going to an investment cast factory. And when we were going up the road, I noticed that it was dirt and also a lot of potholes. And India has a very strong monsoon season. So we were talking, we were like, well, how is a container going to get up this hill when it's the monsoon season? Because it's going to be muddy. So even like simple things like that, it's very important to know the factory, the layout and also the surroundings of the factory. So yeah.
Ben: Yeah. I think, you know, it's certainly helpful to have an advocate who has boots on the ground and does the hard part of weeding out the duds for you so that you can have confidence in placing your project in India and take advantage of all the, you know, advantages they have for low cost and high quality that certainly does exist.
Casey: What about Thailand?
Ben: Yeah. I mean, I think I'll pass this one over to Alex in a second because of course he has a factory here. Our experience in Thailand is that generally the communication is quite good. The level of English is certainly good, and the quality tends to be right at expectation. So, you know, it's more of a market where people do what they say and say what they do.
Casey: Good infrastructure.
Ben: Good infrastructure too. You know, some of the challenges are just benchmarking against those kind of target costs can be a bit of a challenge with, you know, a relatively higher GDP than surrounding markets. But, you know, it's a good place. I mean, Alex? Right.
Alex: For me, yeah, it's...our factory in Thailand, we rarely see power outages. So the infrastructure is pretty decent. And around the surrounding area, the roads, logistics is very convenient. You can have third party logistics and DHL like or just some like large FCO transporters like everything is in place, but there are some issues, for example, in some RFQ, right? Some parts might not just be casting like for casting, we can just use our like molding machines, and everything is in-house, but sometimes they are like forging parts or like a rubber parts. And then I have to go find suppliers in Thailand, right? And sometimes they kind of reply quite slowly, sometimes. Yeah. Or they don't really have to follow up from phone calls and maybe take some time. Eventually they will quote yes. But it takes some time in the requesting for the RFQ stage. Yeah. And the pricing sometimes is, sometimes our price, our casting parts, machining parts is in targets. It's right for right under the target price. Right. But the accessories, like the parts, stamping parts and a lot of different smaller parts that in the assembly with the casting. It's too high. Right. Yeah.
Casey: We've actually seen, it's kind of funny, like in India everyone says yes, but in Thailand it's very hard to get people to a lot of times to take a project because when they decide to quote and take a project, they do a very good job. But a lot of the times getting them to quote, they're very hesitant, a lot of Thai factories. Once they do it, they'll do it very well. But getting them to do it is sometimes tricky. Yeah. And I guess the third country. What about Vietnam? What do you see? Yeah.
Ben: Vietnam is definitely emerging. A lot of, you know, multinational companies are setting up factories there for both finished goods and industrial parts. In general, it kind of, I would characterize it as kind of being in the middle of the two countries we just described in terms of, you know, generally being a little cheaper than Thailand and generally being a little bit more transparent and communicative than India. Like we touched on earlier, you know, there's a little bit different offerings in terms of the manufacturing processes there. There's fewer heavy industry options like forging or casting. It's more injection molding or machining generally. There are some some larger old forges in the north that, you know, the other challenge with Vietnam is it is kind of still, you know, it's geographically it's a little bit of a challenge, because in Thailand you use kind of one central port no matter where anything is being manufactured. But in Vietnam, there's a main port in the north and a main port in the south, and so that can make things like consolidation tricky. It can also make things like subcontracting, you know, if your coating supplier is in the south, but your foundry's in the north, it's a lot of roadways to cover. And the infrastructure is not amazing for facilitating that yet. There's some changes in the works. High speed rail from the north to south of Vietnam, we're looking forward to. But yeah, it's a geographical challenge.
Casey: I think, we've talked to a lot of customers in the US and when they fail in Vietnam, it's a lot of the times, because they don't have a team there, because the English level in Vietnam is not so good. So it's really critical to have a good team in place there and a local team, a local Vietnamese team there to manage the factories. So yeah, we're we're lucky to have a good team in Vietnam to help us with that. Alex is actually one of our manufacturing partners, and we've worked with Alex on a few different projects. Ben, why don't you tell them about one of the projects we did and how we helped consolidate and save our customers some money? Yeah.
Ben: I mean, I think, it's the most recent project we did where we were producing parts that are ultimately used in machinery, and that machinery is specific for the braided hose industry. So we're supplying parts to a manufacturer in the United States who assembles those parts and turns them into a product that's then used all over the world. It's quite a precise product with a lot of challenging tolerances and engineering requirements. We worked with Alex and his company, Maesot Heavy to produce the parts and, you know, that's super helpful. Being that we're all locally based here, it's very easy for us to go on site to communicate in our own time zone, and to, you know, go up and inspect the goods, make sure that everything is according to the print and drawing requirements, and ultimately, we were able to help this particular client by consolidating with another foundry that they work with, with a different style investment casting foundry. And we were able to consolidate and save a lot of shipping costs. Shipping costs are are always kind of fluctuating, rising and falling. But, if you are able to consolidate it, you can create more frequent shipments that are more efficient. They just have take up. You can use the cubic meter allotment a lot better. So we were able to do that. And, that was extremely helpful for our customer because of the nature of their business, they produce a large mix of products at quite low volumes. And so it's important for them to support their just-in-time inventory to work with MOQs that make sense, and we were able to offer savings on logistics by consolidating with another process of casting.
Casey: What immediate actions should companies consider to avoid potential pitfalls in their relocation efforts?
Ben: Yeah, I would say, you know, choosing the right partner is critical. We have countless examples from our clients, horror stories where they've chosen the wrong partner, and, you know, depending on where you're going, it could be anything from like, you know, most of them are pretty good to it's a total crapshoot. And 99% of them are pretty bad. I think there's, Casey you and I have stories of being in India, to meet a prospective vendor that we felt really good about, and had been chatting with online for weeks. And, you know, we pull up to the shop and we know from the second we arrived that it's just not going to be a fit. And we, you know, we just tell our driver to put it in reverse and head on to the next meeting because, you know, it's tough to know who the right partner is. That's why we feel it's important to have the local presence. Go ahead.
Casey: Well, yeah, I was going to say, I think the reason Alex is a really good partner and we've seen this especially in Thailand. Some of these factories, they've been around for decades and some of the children are running the factories now. You know, their dad's given the reins and the children are not really hungry for business. So, Alex is great because, you know, you're very hands on. You know, you're at site, and, you know, when you're partnering with someone, it's really important to to partner with a factory owner that, you know, is hands on and and knows everything about, you know, their operation. Yeah.
Ben: That that ties straight into like, you know, we always talk about in the office about the three Ps. And, you know, the Ps are, they're 'Process', which is, you know, all of your manufacturing infrastructure, your documentation, your quality programing, which is super hyper critical. Then there's 'Price', which is important to every importer, and then but the last one is the 'People', and you need to have people who are flexible, who are willing to solve problems, to dig deep and investigate. Maybe it's not something they've done before, but, willing to study it and, you know, run a feasibility study, see if it's going to be possible, try to find potential solutions to be able to turn these concepts into cast goods or forgings or anything like that. And I think, the three Ps, the 'People' is really important. All three of those are very important. But the people is one you definitely can't miss. Yeah.
Casey: And like going into like, you know people it's hyper critical like especially you know, communication is of the utmost, you know, importance and you know, just being able to, you know, just work always on WhatsApp with, you know, all of our partners, you know, seeing what's going on, you know, send us a picture or we have to go, you know. So yeah, having a good personnel at the factory is very important. Like Alex, when you're working with companies like ours. What do you see as important to partner with a company such as ours? Right.
Alex: For us, the number one quality that we hope that we can get from our customer or partner would be like be very clear on the specification from the start. Because, like from day one, it would be the RFQ. Right. Very first step, RFQ.
Casey: And what needs to go into the RFQ to make it clear? Right.
Alex: So in the RFQ we need the drawing, the material, the manufacturing process. What kind of QC standards are we talking at. Because that will all affect the price. And some customers, they are either unsure or they don't have the right person on this as the coordinator. So it's kind of frustrating for us. Like, okay, so what kind of quality are we talking about? What kind of process are we talking about? Because we have to write the code with our company name on it at the end of the day. So it's really great working with Align Manufacturing too, like from the emails and the information and the communication is very clear that we know from there what we are working with. If Align Manufacturing, if you guys did not get the information from the customer, we immediately get the feedback. Usually Align will contact the customer and get us that feedback and have been really, really helpful.
Casey: Yeah, I think like, what we found is like, you know, working with, you know, factories and guys like you is like it evolves into more of a like we're on the same team. You know, we all have the same goal. So it's important having that clarity. Yeah, so I mean you know we always try to stress to the OEMs or customers in America, don't wait until November for the election. Like, let's like you should start now. You know, like, let's get going. Why is that these OEMs need to move quickly?
Ben: Yeah. I mean, I can understand why people would want to wait and see. This election it involves importers more drastically than probably any other election in history that I can think of. And so there's a tendency to want to wait, but I think that's a counterintuitive tendency. And that's for a few reasons. I think the biggest reason is that there's only a fixed number of factories. And when you look at that cross-section of factories and talk about which ones are actually good, it's a very, very small percentage and capacity at those factories is filling up. And they're filling up from all the first movers and everybody who already moved. And so, you know, importers who are making the switch now, they're frankly, a little bit late to the game. It's not too late, but the longer you wait, kind of the worse your options will be. And you don't want to be stuck with the wrong manufacturing partner. And, you know, on the flip side, the same point of that is, you know, pricing follows, pricing is the same anywhere, it follows scarcity. And so as capacity decreases, price increases. And you better believe if you're bidding, you know, if Trump wins and a 60% tariff is enacted, your factories are going to know that. And when they're pricing out your quotes, they're not going to be pricing out according to benchmarking your 2017 China price. They're gonna go ahead and tack on an extra 60% to that knowing that they're still competitive. Ad so, you know, moving now is very advantageous for importers to get the correct manufacturer and the correct price. And for those who, you know, want to wait and see, that's a game that any, any business is allowed to play. But it's kind of a 50-50 at this point. And, it's like going to Vegas and betting on red. It might be fun for a weekend, but it's probably not something you should do with the future of your business, especially if it's a product focused business.
Casey: And I think, especially like you're looking at timelines, you know, I mean, how long does tooling take usually at your shop, right.
Alex: Um, for the complicated one, it depends on what you do. We we are foundry. Right. So we have the pattern and then the if it's a complex with a cavity and or a different like undercut geometry, we have to have the core box. Right. So if it's just one model maybe, maybe right now we're kind of busy. So maybe around two months for the medium to simple one. Right. But if it's really complex with like multiple multiple core box, many cavities then maybe it's maybe, like, maybe three months. Yeah.
Casey: And that's the thing. I mean, that's that's just the tooling. And then we have to make the sample and then the customer has to approve the sample. And, you know, sometimes the T-Bone sampling doesn't always get approved. So you're just moving the timeline. And so it's yeah, it's really important.
Ben: And there's third party testing and a million variables that can push that timeline out. And so, you know, even if you were to start today, you're looking at, you know, first production shipping out around the election anyway. So um, it's a, you know, it's just kind of, uh, urgency and expediency is is advantageous in this situation.
Casey: We talked about the 301 tariffs, but like what are some other reasons why manufacturers need or should be moving out of China?
Ben: Yeah. I mean, we kind of touched on this. But you know, when we talk about these other factors besides the tariffs, these are what we're driving the large corporations out of China in the first place, before the trade war and before the pandemic. And there's a lot of them. There's a lot of macro trends that are leaning away. There's increasing cost of labor in China as the economy develops there. The cost of labor is is increasing. And of course, that affects the bottom line in these very labor heavy industries. There's an aging workforce associated with the demographic changes in China. And so that's going to reduce the availability of factory workers who are interested to work in the manufacturing industry. And then there's, you know, kind of the red hot one that nobody really wants to talk about. But it's, you know, in the event that kind of what this kind of cold tit for tat trade war that's going on, if that escalates into something hotter, you know, where there's a real conflict, whether it be, something with the US or something with Taiwan, something in Hong Kong or all of the disputed South China Sea territories. You know, all of these are kind of red hot button issues. And if a conflict goes from cold to hot, you can pretty much kiss your supply chain goodbye. And it doesn't matter if you're willing to pay the tariffs at 30% or 60% or 100%, you just won't be able to get it. It'll be like the beginning of the pandemic, but it lasts forever.
Casey: I think it's important for us to say as well, like we're, these are all like facts. We're not against China at all. I mean, I used to live in China for a few years. I really like the Chinese people. But I think the point of this conversation is to really highlight the need to get out, at the very least.
Ben: Yeah. And I think it's, you know, something that's often overlooked or just not always talked about is that the US has placed China as a designated US foreign adversary, and that's a very short list. There's only seven countries on that list. It's North Korea, it's Iran, it's Cuba, it's Venezuela. And China is on that list. And when you look at the trade between the United States and these other countries that have been designated as an adversary, it's virtually nothing. We have strict embargoes against all of these countries. We're not allowed to import anything. It's not a matter of duty. It's just you can't get it. And the fact that the US government has placed China on this list, and most recently was said that it's actually the most potentially dangerous foreign adversary on the list.
Casey: But yet it's our biggest trade partner.
Ben: Our largest trading partner kind of knocked some reasoning behind this policy, which not everyone kind of understands the why or the how, but, you know, that's kind of the why. And the US government is interested in making sure that they can pursue their foreign policy interests without affecting US businesses. And, you know, US businesses would benefit from falling in line with that. The US government is going to keep these in place as long as China is on that list. And yeah, the government wants you to divest.
Casey: The last sort of question I would have for you guys is, you know, how do you see, like the landscape of global manufacturing evolving, you know, as this relationship between China and the US is decoupling?
Ben: Yeah. I mean, I would say, a lot of the growing pains that importers are having now come from working with less developed, less established factories that are not as familiar with the documentation requirements or the expectations and standards of the importers. You've got to remember that when China first started out, people didn't have very nice things to say about Chinese quality or Chinese suppliers. And that changed. And I expect the same thing to happen in our markets. As you know, these factories become more accustomed to working with Western importers. And, I think that some of those growing pains will change. I think we'll see new types of factories emerge as well, where right now we have mostly process-based factories that, you know, it might be a foundry, it might be a forge, it might be a machine shop. But those generally create parts and not products. And when you're talking about a packaged consumer good or even a complex assembly of an industrial good, that's a different kind of factory. That's an assembly factory. And we are seeing those emerge especially for joint ventures that are, you know, getting set up across the region. But I think you'll also see that as an independent job shops for contract manufacturing.
Casey: Yeah, that is a good point. I think, you know, a lot of the times you go to, you know, you go to a Costco or a Target and you, you see, you know, just as you think it might be so simple, but assembly is just it's extremely complex. And setting up an assembly in a factory, it takes time for it to evolve and be mature. So, yeah. Well, do you guys have anything else that you'd like to add into the conversation?
Ben: I, yeah. I mean, just on your last point, you know, other changes, I think you could see, some more friendly trade policies. You know, India has really been the shining star of this, to really recognize this opportunity and seize it with really manufacturing forward policies. The Made in India policy, which grants, you know, interest free loans as well as all kinds of tax incentives for manufacturers. I think we'll see that deployed across the region and in India. We'll see them continue. Right. Ease of doing business. I see it improving where there may be, you know, importation of child parts to be assembled or the allowance of purchasing a child part domestically. But having it be VAT exempt because it's ultimately being exported. So I think we'll see some change in the regulatory environment in these countries, which are catching on to the opportunity that's there. And it's just it really is a massive opportunity.
Casey: Yeah. I really appreciate you guys joining me. It's been a really nice conversation. Alex came all the way down from north Thailand to join us on this podcast. So Alex, thank you very much for joining us. Thank you for joining us today. If you found this discussion insightful and are considering how best to navigate the complex manufacturing shifts, I encourage you to read our recently published report Guiding US-Based Manufacturers Out of China. It's packed with in-depth analysis, real-world case studies, and practical strategies to help you make your informed decisions. To discuss your specific needs, don't hesitate to get in touch with us at Align Manufacturing. Together, we can ensure that your transition is smooth and successful. Thank you again for watching and we look forward to helping you secure your manufacturing future beyond China.
For a more in-depth exploration of these themes, download this Align Manufacturing report:
Innovations Transforming Investment Casting
Investment casting, also known as lost-wax casting, has existed for centuries in manufacturing. This is a process for casting metallic parts with great precision and detail; it is, however, a somewhat old format compared to newer versions. This article takes a look at some of the recent developments in investment casting today, with a special focus on 3D printing and new material technologies.
Lost-wax casting is a manufacturing process that produces a wide variety of products of complex geometries. It generally involves a wax pattern surrounded by a ceramic shell. When the shell hardens, the wax is melted out to leave a mold. Hot metal is poured into the mold and the casting can be completed. It is highly commended for products that have excellent surface finish quality and have close tolerances. It is applied in several industries, from aerospace and automotive to medical, where precision, quality control and automation take the foremost place.
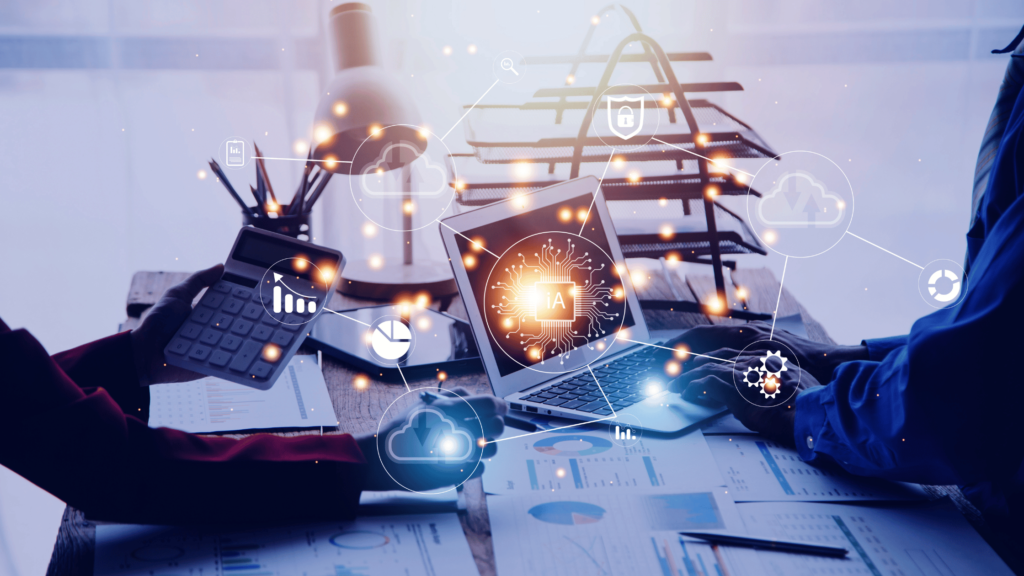
Historical Context and Traditional Methods
Historical origins date back over 5,000 years to ancient civilizations like Mesopotamia and Egypt. Traditional investment casting usually involves several labor-intensive steps:
- Pattern Creation: A wax pattern has to be made that is a replica of the final part.
- Assembly: Several wax patterns can be attached to a tree-like configuration.
- Shell Building: The wax pattern is to be dipped into a ceramic slurry and coated with sand to produce a hard shell.
- Wax Removal: The shell is heated to melt and drain out the wax to leave behind a hollow ceramic mold.
- Casting: Molten metal is poured into the mold.
- Finishing: The ceramic shell is broken away from the cooled metal and the metal part is finished.
While effective, this traditional method is time-consuming and requires significant manual labor. Innovations in technology are addressing these challenges and transforming the process.
3D Printing: A Game Changer in Investment Casting
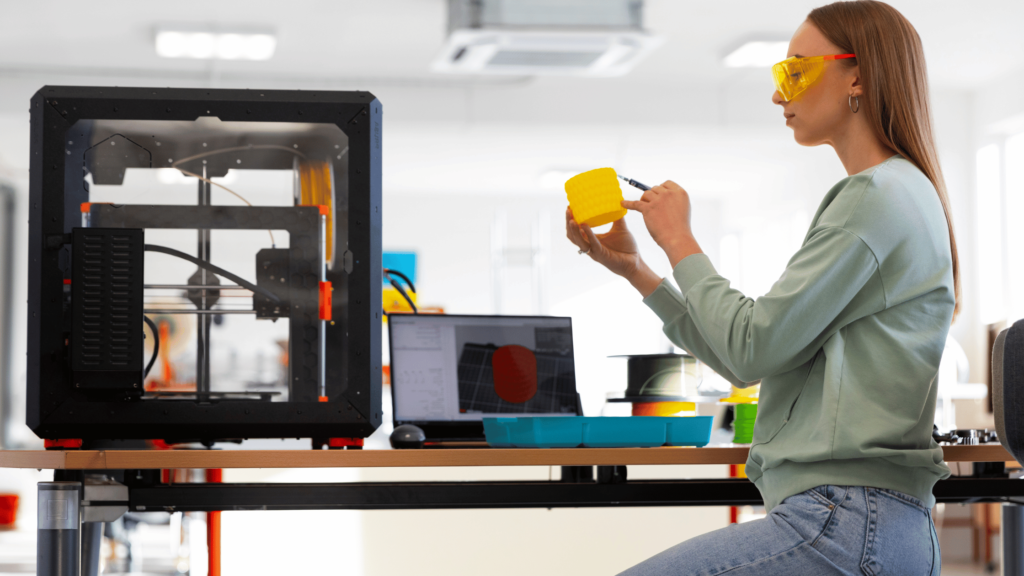
Application of 3D printing or additive manufacturing in the investment casting has significantly revolutionized the process. Earlier, the wax patterns were made by molds and were laborious and, on occasion, costly, especially for something with complex shapes. 3D printing avails a cost-effective and quick alternative.
Advantages of 3D Printing in Investment Casting
- Design Flexibility: It is perfect for creating complex geometries, impossible or very hard to create using traditional methods.
- Reduced Lead Time: The application of 3D printing to make patterns nullifies the need to make the molds, and this way, it reduces the time taken to manufacture patterns significantly.
- Cost Effective: In case of low volume production itself, or prototyping, 3D printing can turn out to be more cost-effective than the traditional lost-wax method in cases of tooling.
- Parts Customization: it has easy parts customization. No additional tooling, neither molds are needed.
3D Printing Technologies in Use
There are several 3-D printing technologies applied to investment casting, each with its unique sets of benefits:
- Stereolithography (SLA): This makes use of a laser to cure liquid resin into solid shapes. It can come up with never seen before detailed and accurate patterns.
- Selective Laser Sintering (SLS): It is a laser kerosene that fuses powdered material, layer by layer, to make powerful and robust patterns.
- Fused Deposition Modeling (FDM): In the FDM process, the melted material gets extruded and laid down to construct the layers. The cost-effective and rapid method to build patterns which are relatively simple.
New Material Technologies
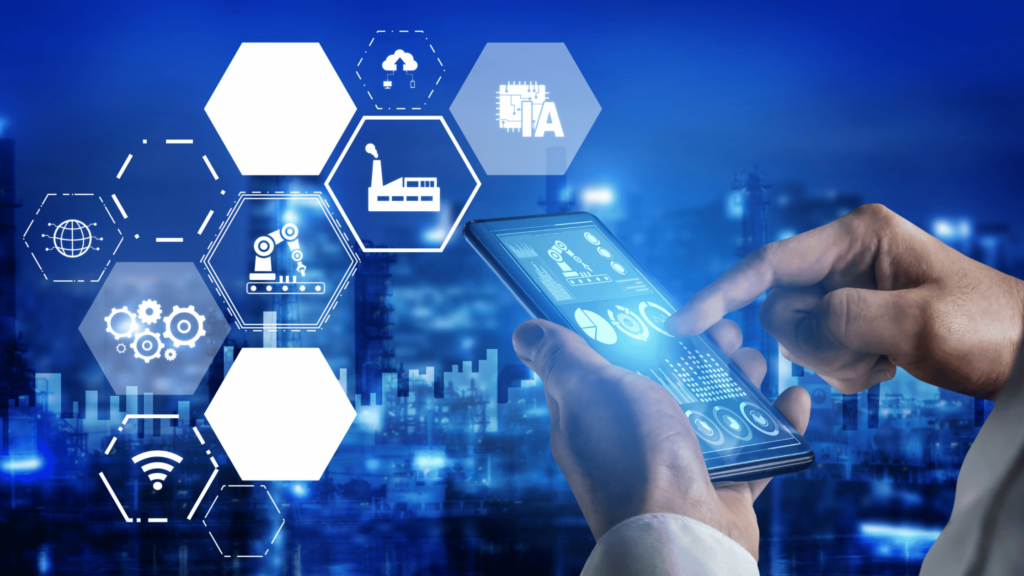
Advancements in material science are also revolutionizing investment casting. New material and new alloys are making the cast parts of better quality, robust, light, and durable.
High-Performance Alloys
New high-performance metals are manufactured to cater to the demanding needs of industries ranging from aerospace, automotive, and medical. These new materials boast improved mechanical properties, corrosion resistance, and temperature tolerance.
Ceramic Shell Improvements
Advanced ceramic shells revolutionize the investment casting process. Improved ceramic formulations enhance the strength and stability, thus reducing the risk of defects and increasing the quality of the cast parts.
Benefits of Advanced Ceramic Shells
- Higher Strength: Improved ceramic materials can withstand higher temperatures and mechanical stresses, allowing for the casting of more robust parts.
- Reduced Defects: Enhanced formulations reduce the occurrence of common casting defects such as cracks and inclusions, leading to higher quality parts.
- Faster Production: Advanced ceramics can shorten the shell-building process, increasing production speed and efficiency.
Integration of Smart Technologies
The integration of smart technologies, such as the Internet of Things (IoT) and Artificial Intelligence (AI), is also making its way into investment casting.
IoT and AI in Investment Casting
- Real-Time Monitoring: The Internet of Things will monitor the casting process in real time by means of different built-in sensors to provide data for maximizing production and minimizing defects.
- Predictive Maintenance: Using AI algorithms to analyze, equipment breakdowns could be predicted, and timeously fixed before they occurred. Reduces downtime and maintenance costs.
- Process Optimization: AI can analyze production data and detect inefficiencies. It can even suggest alternatives, enhancing the general efficiency of the process used in casting.
Future Trends
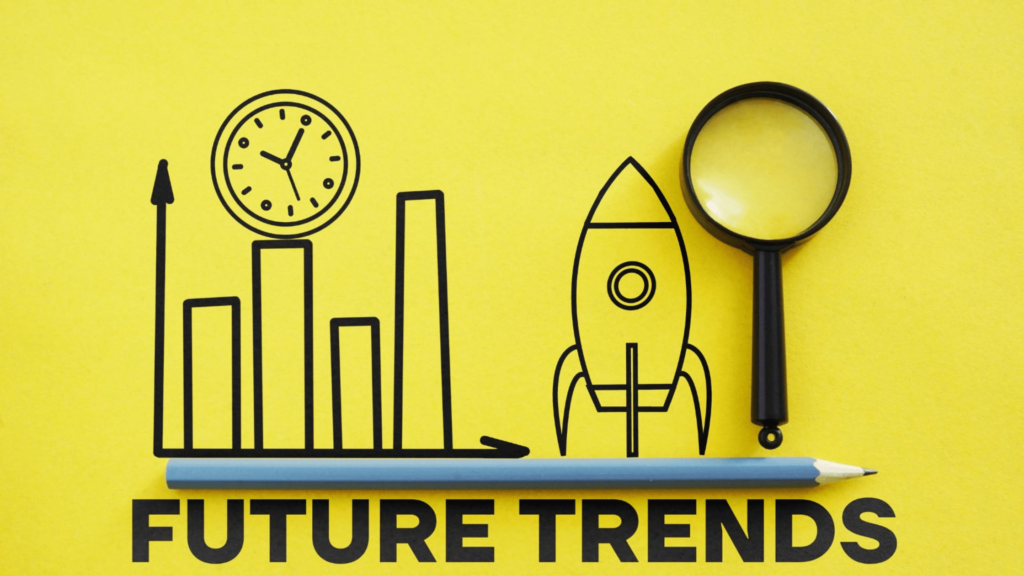
The future of investment casting looks promising, with continued advancements in 3D printing, material technologies, and smart manufacturing. As these technologies evolve, they will further enhance the capabilities and applications of investment casting, opening up new possibilities in various industries.
Emerging Technologies
- Digital Twin Technology: This is the technique for the development of a digital replication or model of physical process operation. This helps to monitor internal operations in real time for optimization. In general, it can help in the prediction and prevention of potential problems that would occur.
- Sustainable Practices: Developments of materials and processes are also done in sustainability. A conductive approach towards eco-friendly materials and the recycling of the materials used in the casting is reducing the impact of investment casting on the environment.
- Hybrid Manufacturing: Traditional techniques used in casting are combined with modern-day techniques, like 3D printing, to design hybrid manufacturing processes. This develops efficient production and better-quality parts.
Conclusion
Investment casting is indeed being transformed through innovative technologies. Other applications of 3D printing and new material technologies besides improving efficiencies and qualities within the manufacturing process continue to expand the applicability of the casting process. These innovations will undeniably be the logos of the manufacturing future that will thus make the investment casting process more flexible and effective than ever before.
Automation and Quality Control in Investment Casting
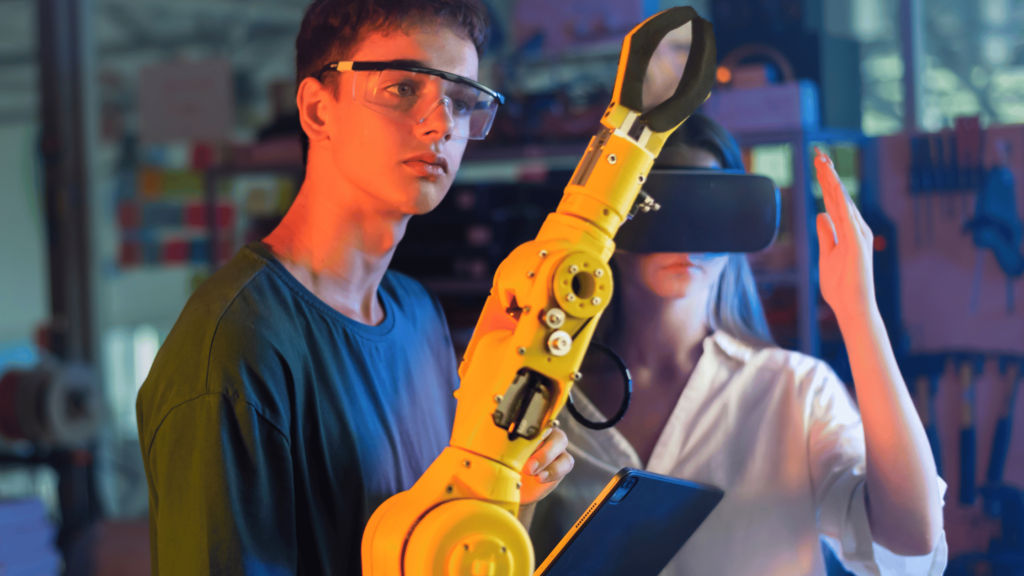
Investment casting, also known as lost-wax or precision casting, is one of the oldest doctrines to intricately craft components. Though the origin of the process can fold many centuries back, it does not necessarily mean that it is antiquated thereby. The improvements and changes made have been brought on by automation and advanced quality control. These have improved the areas of efficiency, precision, and part reliability, all of which contribute to the competitiveness of investment casting in this high-paced manufacturing environment. This blog goes through the aspects of automation: its role in the enhancement of the process and the critical quality control measures that ensure part reliability.
The process of Investment Casting
Investment casting is a manufacturing process for generating complicated and high-precision components. Such a process starts with the creation of a wax pattern, which is an exact replica of the desired part. The pattern is then coated with a ceramic shell to fashion a mold. After this ceramic shell hardens, wax is melted out of it, leaving a cavity that is then filled with molten metal. Later on, after it solidifies, it is to be broken away to reveal the final casting. The process is marked by its ability to generate parts with high surface finish and tight tolerances, which qualifies it to be applied in critical industries such as oil, railway, construction, and industrial sectors.
The Role of Automation
Automation has revolutionized many aspects of the lost-wax casting process. Manufacturers no longer have to rely on the human hand for repetitive and precise tasks. Instead, automated systems have been incorporated, which allow them to achieve higher levels of precision while reducing lead times and improving overall efficiency. Outlined below are some of the ways in which automation is bringing much improvement to precision casting.
Automated Pattern Creation
Historically, the making of wax patterns has been a very labor-intensive process. However, this process has been improved with automation to a great extent. Automated pattern making machines can produce wax patterns with remarkable tolerance and repeatability based on computer-aided design data.
- Faster Delivery and Shorter Production Time: Automation processes the pattern making at a quicker rate, thus reducing the lead time for production.
- Makes Patterns of Complex Geometries: Machines are capable of producing complex geometries for the patterns of designs that would not either be possible or practical to reproduce using manual techniques.
Robotic Shell Building
Another area of the process that has been revolutionized with automation is the shell building process in making the ceramic shell around the wax patterns. The robotic system carries out the wax pattern’s dipping, draining, and stuccoing (coating with ceramic).
- Higher Precision: Automation ensures precise control over the amount and parameters of pouring. Since molten metal is poured into ceramic shells, automation can maintain the right temperature of molten metal thus preventing defects due to poor pour temperature.
- Even Pouring: Delivering molten metal through robots at a steady pace prevents defects like entrapment of air or incomplete fills.
- Safety: Since automation prevents accidents due to molten metal handling, the workers’ safety is ensured.
Quality Control Measures in Investment Casting
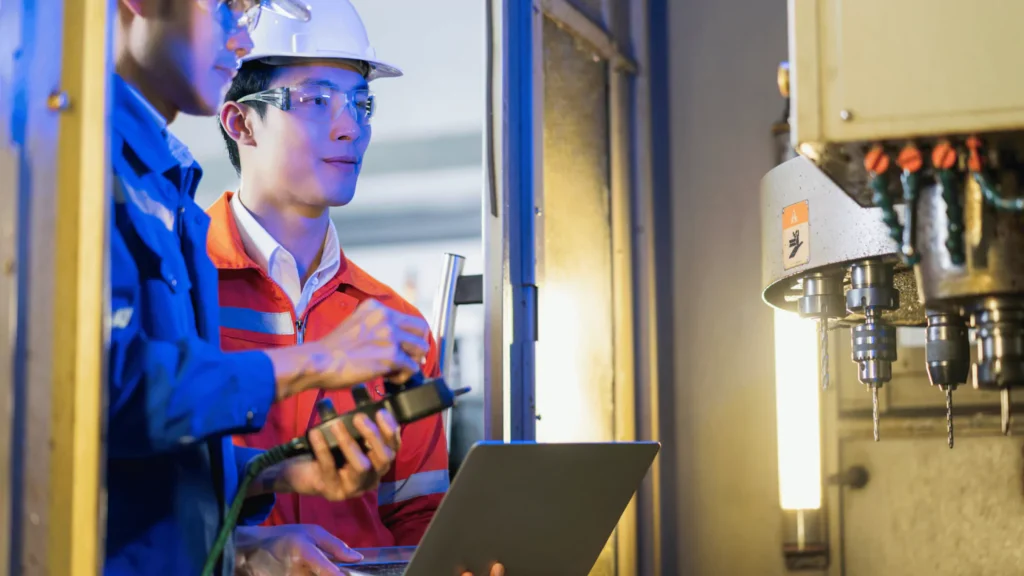
Quality control involves various critical steps, from inspection of patterns in the initial stages to the final evaluation of the products. In a nutshell, the following is major quality control measures in lost-wax casting:
Pattern Inspection
The quality of the final cast part depends on the tolerance of the wax pattern. Inspection of the wax pattern is hence an important step.
- Dimensional Criteria: The patterns are gauged for dimensional features, and modern gauging appliances like CMM are used for inspection.
- Surface Condition: Scrutinizing the surface of the wax pattern for imperfections prevents flaws from appearing in the final casting.
Shell Integrity Testing
This is critical in the case of the ceramic shell used in the casting process. Any defect in the shell may result in defects in the final part.
- Visual Inspection: The shell is visually inspected for cracks, incomplete covering, or some other defects.
- Non-Destructive Testing (NDT): Ultrasonic testing and radiographic inspection help to detect internal defects in the ceramic shell.
Metal Quality Control
The quality of the molten metal is another important factor in casting. Quality metal is free of impurities and has the right composition to produce quality cast parts.
- Spectroscopy: This will be applied in analyzing the chemical composition of the metal to assure that it meets the required specifications.
- Inclusion Analysis: The analysis is carried out using such techniques as optical and scanning electron microscopy to detect non-metallic inclusions present in the metal.
Post-Casting Inspection
After the metal has been poured and the ceramic shell has been removed, these post-cast inspections help ensure the quality of the end product.
- Dimensional Testing: Checking the dimensions of the cast part to verify with the specifications undertaken.
- Surface Testing: Testing the cast part for cracks, porosity, or surface roughness.
- Mechanical tests: Test and confirm that mechanical properties, including, for example, tensile strength and hardness, are as per or meet the specified in the required specification.
Advanced Automation Techniques
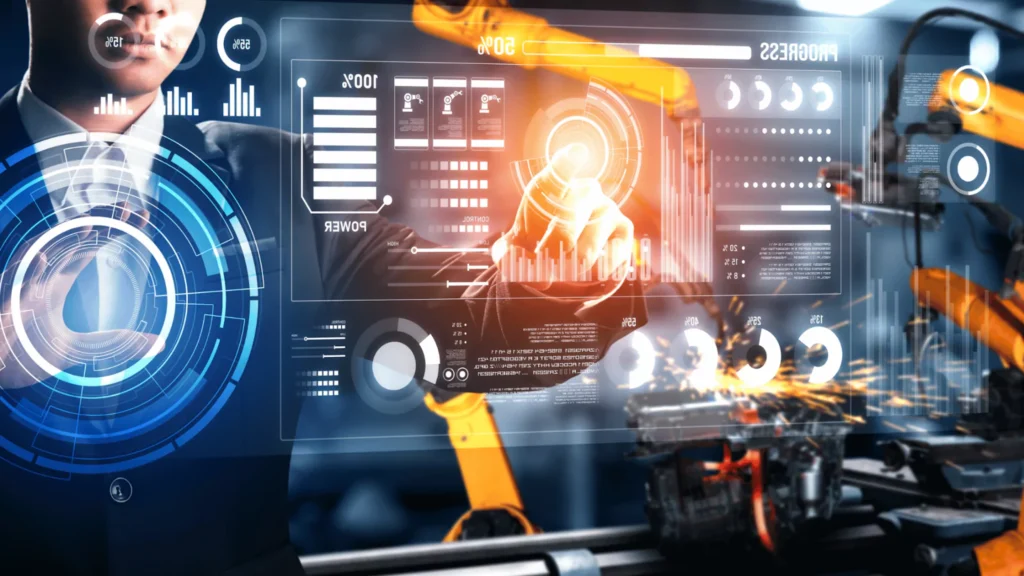
The adoption of advanced automation further elevates the process. Artificial intelligence, machine learning, and the Internet of Things are some commonly used concepts.
Artificial Intelligence and Machine Learning
In the context of casting, artificial intelligence and machine learning can be harnessed to study the huge data that comes out from the casting process to note the patterns and sometimes even predict results. The manufacturers employ it for improvement in process and quality control.
- Predictive Maintenance: Predicts when equipment is likely to fail, allowing maintenance to be planned in advance, thereby reducing unplanned downtime.
- Process optimization: ML algorithms can analyze process parameters and suggest adjustments which enhance yield and reduce defects.
- Defect Prediction: AI can predict the likelihood of defects in cast parts and allow early intervention and corrective measures.
Internet of Things (IoT)
IoT is about implementing a setup to help collect data regarding various parameters around the casting process. IoT sensors monitor the temperature, pressure, and other critical parameters of the casting process in real-time. Data collected by IoT can further be analyzed for trends, detecting anomalies, and optimizing processes. This process can be adjusted and controlled remotely, allowing for interventions from a distant location.
Automation and Innovation in Critical Industries
Automation and quality control in investment casting have brought transformative changes, particularly in critical industries. These industries demand high precision, reliability, and innovation, and it has risen to the challenge.
Digital Twin Technology
Digital twin technology means a fully detailed virtual copy of the foundry process. The model is digital, allowing for process simulation and optimization in real-time.
- Process Simulation: The digital twins can simulate various situations, through which the manufacturers can optimize process parameters.
- Predictive Analytics: Data from the digital twin is subjected to analytics to allow manufacturers to make predictions concerning possible failures and, in that way, take preventive actions.
- Continuous Improvement: The foundry process can be continually monitored and improved through digital twin technology, thereby increasing process efficiency and quality.
Sustainable Manufacturing Practices
The current trend in the manufacturing industry is a move toward more sustainability. Automation and quality control make possible more sustainable lost -wax casting. An automated process ensures optimization in the consumption of energy and, in return, minimizes the environmental impact brought about by the casting process. Quality control measures will also help reduce defects and scrap, thus reducing waste materials and conserving resources. Material science has not been left behind in this quest, and more eco-friendly casting materials have been developed.
Conclusion
Automation together with quality control has created the most significant changes in the industry of investment casting. With the help of advanced technologies, the manufacturer can achieve the highest levels of precision, efficiency, and part reliability. Automation of casting steps, starting from pattern creation up to pouring, and quality control of the results of every step, streamlines the process, ensuring that every part meets strict standards. Financial investment casting will only shine more with the constant development of technologies such as AI, IoT, and digital twins. The innovations will put manufacturers well in the race in a market that is rapidly changing and in regard to top-quality components meeting high requirements, especially in the aerospace, automotive, and medical industries. It is expected that the merging of automation and quality control will bring further improvements in functionality and sustainability.
The Materials Behind Investment Casting
Investment casting is a widely used manufacturing process that allows the production of intricate and complex metal components with high precision. This process involves creating a wax pattern of the desired part, coating it with a ceramic shell mold, and then melting the wax out to create a hollow metal mold. The mold material is then filled with molten metal to produce the final component.
One of the critical aspects of lost-wax casting is the choice of materials, both for the wax pattern and the shell, as they directly influence the quality, integrity, and performance of the finished product. In this article, we will explore various precision casting materials, including plastic pattern materials, shell materials, and the metals commonly used for casting.
We will delve into their properties and characteristics, highlighting their suitability for different applications and discussing their advantages and limitations in the investment casting process.
Advantages of Investment Casting
Precision casting is a highly advantageous manufacturing process, known for its ability to produce precision components with intricate and complex shapes that other methods may struggle to achieve. One of the standout benefits of this technique is its exceptional dimensional accuracy and the ability to maintain tight tolerances, which are essential in industries such as aerospace and medical devices where precision is paramount.
Additionally, investment casting can utilize a vast range of materials, providing flexibility in application across various industries. The process also allows for excellent surface finish, reducing the need for additional machining and finishing steps, which can significantly lower production costs.
Moreover, the versatility in size and weight of the components that can be produced makes it suitable for both small and large parts, enhancing its applicability for different product requirements.
If you want to know more about investment casting, check out our article about The Basics of Investment Casting: An Introduction.
But for now, let’s dive into the different materials that can be used for the different casting methods.
Stainless Steel
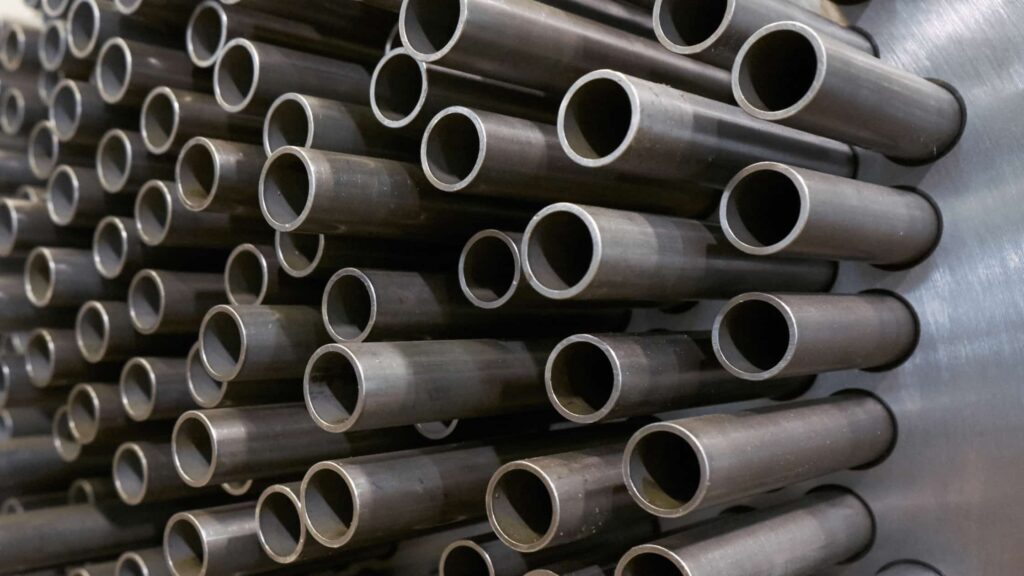
It is a versatile material with numerous applications and advantages. Its primary characteristic is its superior durability, making it ideal for various industries. One significant area where stainless steel is extensively used is investment casting.
In the automotive industry, stainless steel is utilized in gearbox parts and gears. The high strength and corrosion resistance of it ensure that these components can withstand the demanding conditions of a vehicle’s transmission system. Moreover, stainless steel’s resistance to heat and wear makes it an excellent choice for cam components, which are essential for controlling the opening and closing of valves in an engine. Golf club heads also benefit from stainless steel’s durability and resistance to corrosion, ensuring longevity and performance on the golf course.
Compared to other materials, it offers numerous advantages. Its corrosion resistance eliminates the need for maintenance and frequent replacements. Additionally, it can withstand high temperatures, making it suitable for applications where heat resistance is crucial. Furthermore, the aesthetic appeal, with its shiny and clean appearance, adds to its desirability in various industries. Overall, stainless steel’s unmatched durability, corrosion resistance, and versatility make it a highly sought-after material for investment casting process, automotive gearbox parts and gears, cam components, golf club heads, and many other applications.
Low Alloy Steel
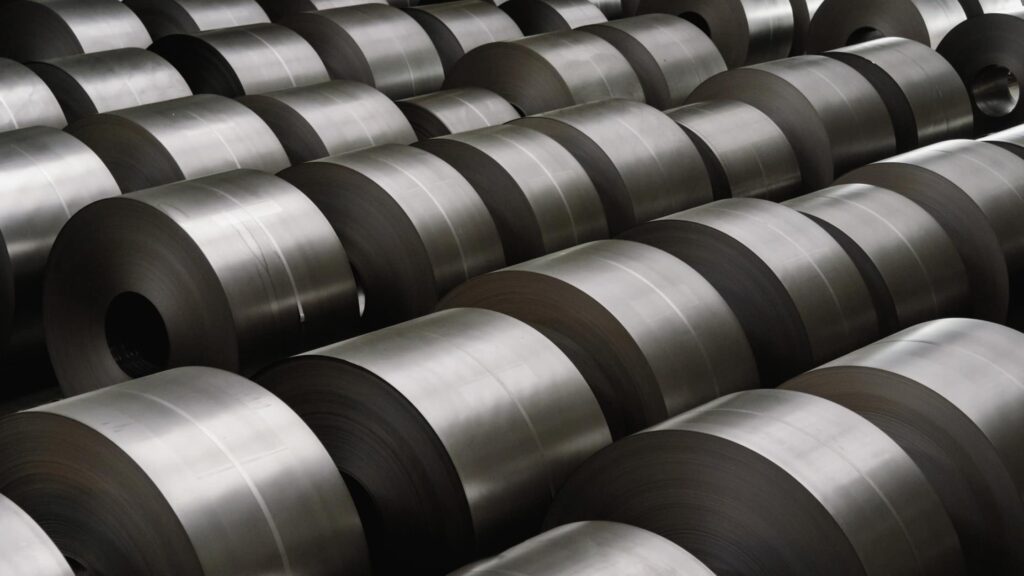
Low alloy steel, as the name suggests, is a type of steel that has a low percentage of alloying elements, usually less than 8%. This makes it more affordable compared to high alloy steels, which contain higher amounts of alloying elements.
It offers numerous benefits due to its beneficial mechanical properties. It has excellent strength and toughness, making it suitable for demanding applications where high strength and impact resistance are required. Additionally, it has good corrosion resistance, making it ideal for use in corrosive environments.
One of the key advantages is its suitability for specialized heat-treating processes. These processes involve altering the microstructure of the steel to enhance its properties. For engineered parts, low alloy steel can undergo specific heat treatments such as quenching and tempering to achieve desired hardness, strength, and wear resistance.
The applications are diverse. It is commonly used in the automotive and aerospace industries for parts that require high strength and durability, such as engine components and aircraft landing gear. Low alloy steel is also widely used in construction, oil and gas, and manufacturing industries for structural applications and machinery components.
Aluminum Alloy
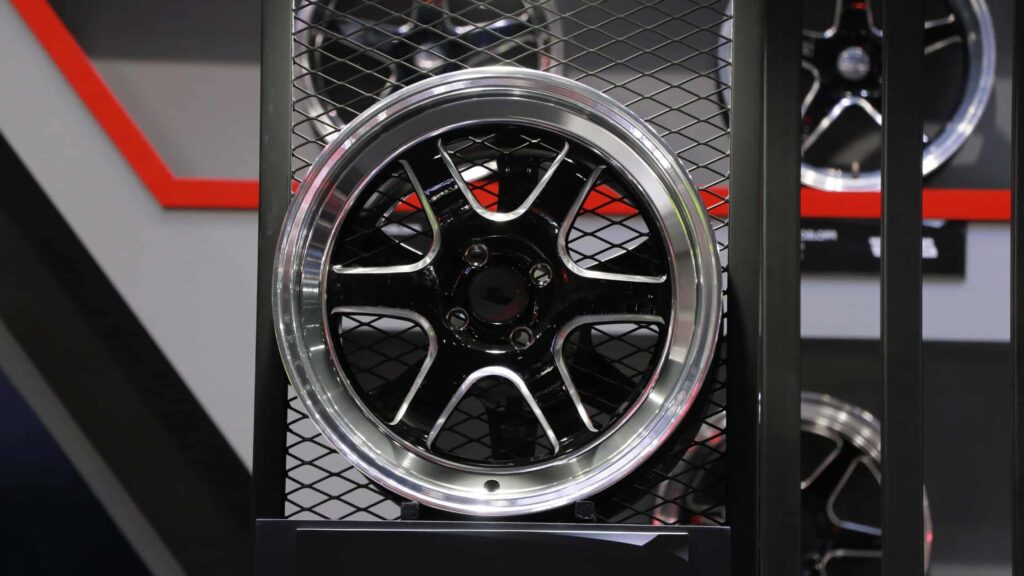
Aluminum alloy is a versatile material widely used for investment castings due to its beneficial properties and wide range of applications. Its key advantages include excellent strength-to-weight ratio, corrosion resistance, and high thermal and electrical conductivity.
Investment castings made of aluminum alloy find applications in various industries, including automotive, electronics, and industrial equipment. In the automotive sector, they are preferred for their lightweight nature, which helps improve fuel efficiency and reduce emissions. They are commonly used for components like engine blocks, cylinder heads, and wheels.
The blend of aluminum, silicon, and magnesium greatly enhances the strength and suitability of aluminum alloy for these applications. The addition of silicon provides improved fluidity during the casting process, making it easier to achieve intricate and complex shapes. Magnesium, on the other hand, increases the alloy’s strength and ductility, allowing it to withstand high-stress applications.
In electronics, aluminum alloy investment castings are used for heat sinks, connectors, and housings, as they possess excellent thermal conductivity, ensuring efficient heat dissipation and component protection. In the industrial equipment sector, these castings are employed in pumps, valves, and machinery components, benefiting from the alloy’s durability and resistance to wear and tear.
Carbon Steel
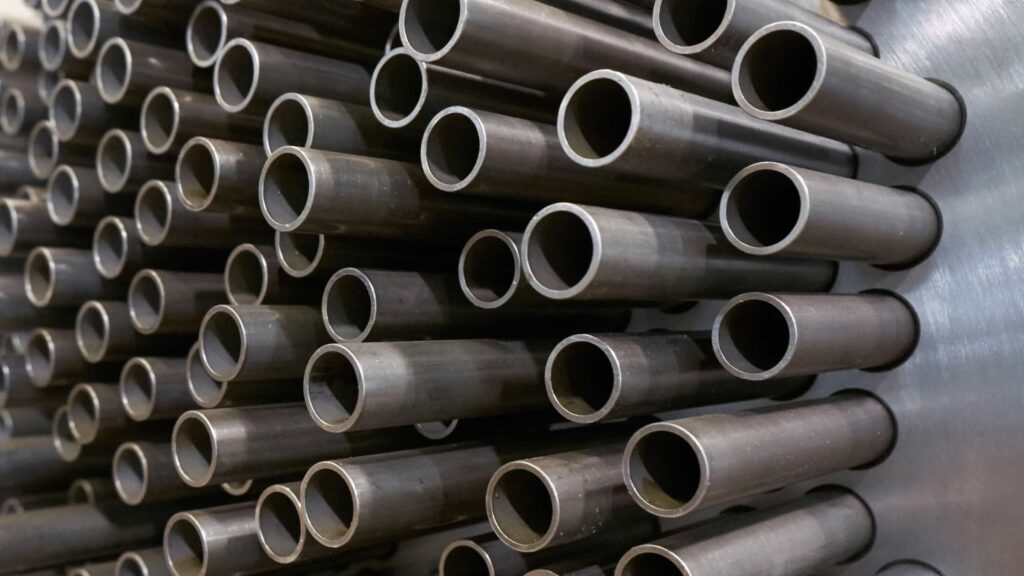
Carbon steel, composed primarily of carbon and iron, is a widely used material known for its low cost compared to other alloys. It comes in various grades based on carbon content, with higher carbon levels resulting in stronger and harder steel.
Carbon steel can be easily shaped and formed into different products, and its performance can be improved through heat treatment, which enhances its hardness, strength, and toughness. One notable property is its ferromagnetic nature, making it useful in motors and electrical appliances. Carbon steel is commonly used in the construction of electric motors, transformers, and generators due to its magnetic properties and cost-effectiveness.
Super Alloy
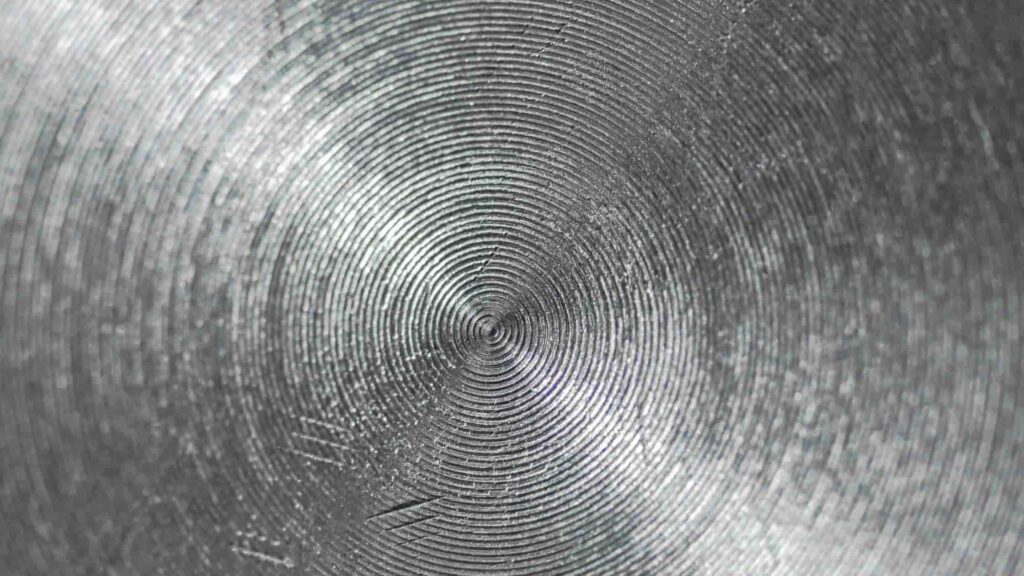
Super alloys, or high-performance alloys, particularly nickel-based and cobalt-based, exhibit exceptional mechanical strength, resistance to extreme temperatures, corrosion, oxidation, and wear. They retain their mechanical properties at temperatures exceeding 1000°C, making them indispensable in gas turbine engines, aerospace components, and nuclear reactors.
Super alloys also offer excellent corrosion resistance in aggressive chemicals, acids, and seawater, benefiting chemical processing plants, marine applications, and oil and gas exploration. Their wear resistance allows them to withstand abrasive materials and heavy mechanical loads, making them ideal for high-stress components like turbine blades and jet engine parts. Despite their higher cost compared to sheet metal, super alloys’ superior properties, reliability, and longevity make them a cost-effective choice for demanding applications.
Copper Alloy
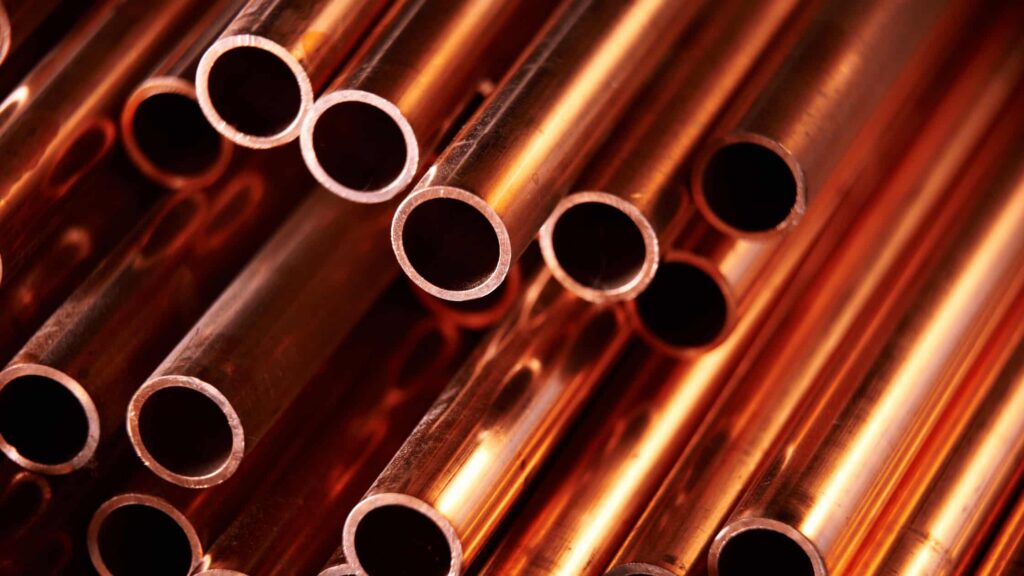
Copper alloys, created by mixing copper with other metals, offer superior strength, corrosion resistance, electrical conductivity, thermal conductivity, and good formability. Bronze and brass are two common types of copper-based alloys used in investment castings. Bronze, an alloy of copper and tin, offers high strength, corrosion resistance, and excellent thermal conductivity, making it suitable for musical instruments, statues, and fittings. Brass, an alloy of copper and zinc, is known for its attractive appearance and easy molding, making it popular for decorative items, plumbing fittings, and electrical connectors. Beryllium-copper, one of the strongest copper-based alloys, possesses exceptional corrosion resistance, high strength, hardness, and electrical conductivity, making it ideal for components in the aerospace and automotive industries subjected to harsh environments and high stress conditions.
Cast Iron
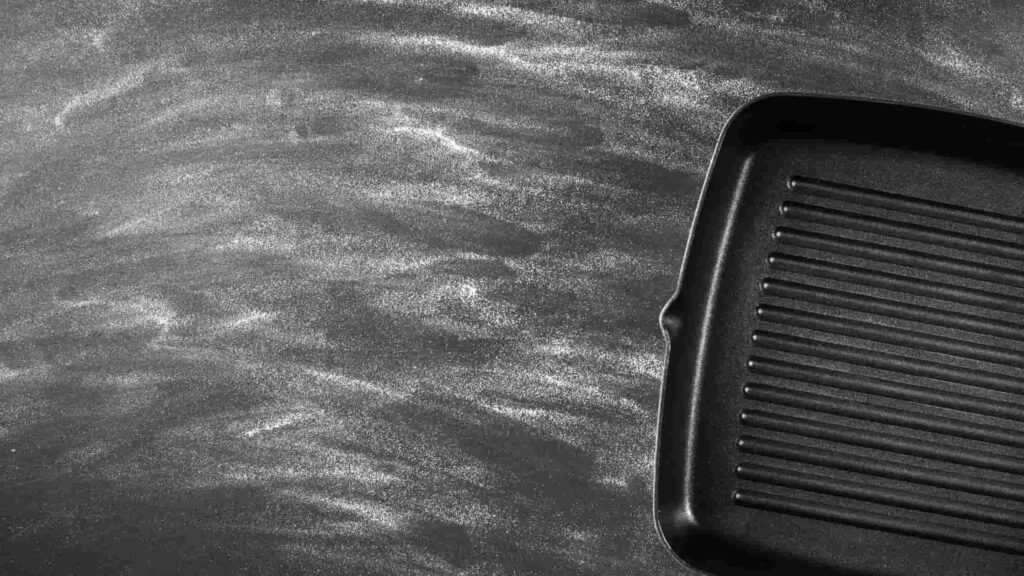
Cast iron is a strong and versatile material that is commonly used in various industries due to its unique characteristics. It is derived from iron and carbon, with a carbon content of at least 2%, making it harder and more brittle than steel. The production process of cast iron involves melting iron and adding carbon and other alloys to achieve the desired properties. This mixture is then poured into molds and allowed to cool and solidify.
One characteristic that makes cast iron desirable is its affordability and high accuracy. Iron castings, especially those made from gray iron and ductile iron, are known for their cost-effectiveness and precise dimensions. This makes them suitable for a wide range of applications, from automotive parts to appliances.
Gray iron, in particular, is easy to cast and has good damping properties, making it ideal for applications that require vibration resistance. On the other hand, ductile iron offers high strength, heat-resistance, and toughness. Its complex production process, which involves adding magnesium to the iron-carbon mixture, results in superior mechanical properties. However, this more intricate process leads to higher production costs compared to cast steel.
Key Takeaways
The selection of metal casting is a critical decision that significantly impacts the final product’s performance and cost-effectiveness. By carefully considering factors like the part’s function, desired mechanical properties, and compatibility with the investment casting process, manufacturers can ensure they choose the optimal ceramic material for their application. This not only guarantees the part meets its performance specifications but also minimizes the need for extensive post-processing, leading to improved production efficiency and tooling cost savings. Therefore, investing time and effort in material selection is a strategic step that unlocks the full potential of precision casting for a broad range of industries.
The Basics of Investment Casting: An Introduction
Investment casting, also referred to as precision casting, is a method for crafting detailed and high-quality metal parts with excellent accuracy and smooth finishes. It’s utilized in sectors such as aerospace, automotive, healthcare, and defense.
This technique offers multiple benefits, including the capability to fabricate complex and intricate designs that might be challenging or unachievable with other manufacturing processes. It also delivers high dimensional accuracy, as the mold faithfully reproduces the details of the die. Additionally, the method allows for fine surface finishes, reducing the need for further machining.
How Investment Casting Works
The investment casting process involves creating a wax pattern, encasing it with a ceramic material, and then heating it to remove the wax, leaving behind a mold. Molten metal is then poured into this mold, which solidifies to form the desired metal object.
Understanding the Step-by-Step Investment Casting Process
Step-by-Step Breakdown:
- Creating the Wax Pattern: The process begins by shaping a wax pattern of the part to be cast. This can be done by injecting wax into a metal mold or by sculpting the wax by hand. The wax model is then attached to wax sprues, which act like channels guiding the liquid metal during the casting.
- Building the Ceramic Shell:The wax pattern attached to sprues is dipped into a ceramic slurry followed by a coating of ceramic particles. This step is repeated multiple times to build a thick ceramic shell around the wax. Each layer must dry thoroughly before the next is applied.
- Melting Out the Wax:Once the ceramic shell is sufficiently thick, it is heated in a furnace. This step melts the wax inside the shell, which is then drained out, leaving a hollow ceramic mold that mirrors the original wax pattern.
- Pouring the Molten Metal:The ceramic mold is preheated to prepare for metal casting. Metal is melted in a crucible and then poured into the hot ceramic mold through the sprues.
- Removing the Ceramic Shell:After the metal has cooled and solidified, the ceramic mold is broken away, revealing the metal part. Sprues are cut off, and any remaining ceramic fragments are cleaned off the metal.
Further Steps to Perfect the Casting:
- Refining Wax Patterns:The accuracy of the wax patterns is crucial as they directly influence the final metal part’s details. Techniques like hand carving, using steel tools, or 3D printing are employed based on the complexity and quantity needed. Each method offers different benefits, from artisanal detail to consistent mass production.
- Setting Up the Wax Assembly:Wax patterns are mounted to a sprue and carefully arranged on a casting board to ensure stability and precise alignment during casting. This setup is crucial for maintaining design integrity and ensuring the molten metal flows correctly into each cavity.
- Forming the Mold Shell:The assembly is repeatedly dipped into a ceramic slurry and coated with sand stucco to form a strong, heat-resistant mold. Achieving the right thickness and strength of the shell is vital for withstanding the molten metal’s temperature.
- Wax Removal Techniques:Removing the wax can be done manually or using automated ejectors in the die, depending on the precision and delicacy required. This step is critical for maintaining the mold’s integrity and ensuring clean metal flow.
- Casting the Metal:Techniques such as gravity pouring, vacuum pouring, or pressure casting are chosen based on the part’s complexity and required precision. Each method has its advantages in achieving a defect-free cast.
- Final Touches on Castings:After breaking the mold, the castings are finalized by removing any excess material and refining the surfaces. This can involve various finishing techniques, depending on the metal and the part’s function.
What Is the Main Goal of Investment Casting?
It has been around for centuries and is used to create complex metal parts with great precision. The process of investment casting starts with a wax pattern, which is coated with ceramic to form a hollow mold after the wax is melted away. The main goal of lost-wax casting is to produce intricate, high-quality metal components that are tough or impossible to make with other methods. This casting process is favored in industries such as automotive, aerospace, defense, and jewelry for its ability to produce detailed and precise components.
If you are looking for a more in-depth explanation about the different materials that can be used in investment casting, check out our article about The Materials Behind Investment Casting.
Advantages of Investment Casting
Investment casting is great for making complex and detailed shapes that would be hard to make with other methods. It’s very accurate because the mold captures all the details of the wax model. It also makes parts with smooth surfaces, which means less work is needed to finish them. Some things made with this method include turbine blades, surgical tools, and jewelry.
Understanding the Disadvantages of Investment Casting Products
Despite its advantages, it’s important to be aware of the potential drawbacks of using this process. Here are some of the main disadvantages:
Size and Weight Limitations:
- Lost-wax casting has inherent limits on the size and weight of the products it can produce. The detailed nature of the process restricts the dimensions and mass that can be achieved effectively.
- This method is not ideal for manufacturing large parts or components that require substantial volumes of common material. For larger parts, alternative manufacturing methods might be necessary.
Extended Production Time:
- The process involves several intricate steps, including creating wax patterns, assembling ceramic shells, and multiple stages of heating and cooling. These steps contribute to a prolonged production cycle.
- This extended timeframe can be a significant disadvantage in industries where rapid time-to-market is crucial.
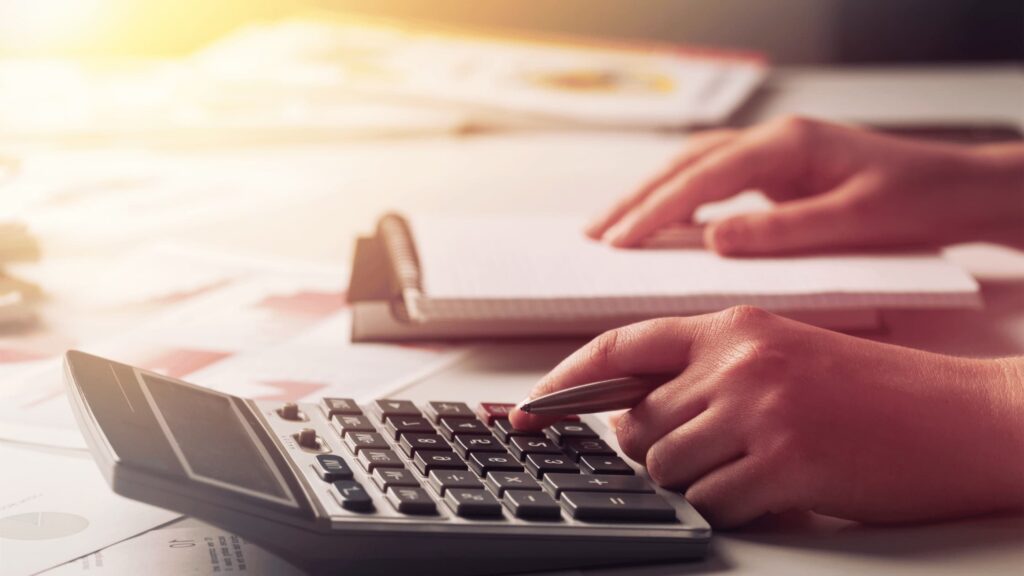
Assessing the Cost of Investment Casting
Although initially perceived as expensive, various aspects of the process contribute to its overall cost-effectiveness.
Initial Tooling Investment:
- It requires significant upfront investment in tooling, including the creation of wax patterns and ceramic molds. This precision work necessitates expertise, which adds to the initial costs.
- Despite these higher initial expenses, the durability of the molds allows them to be reused for multiple production runs, which lead to cost savings per unit over time.
Material Costs:
- The process uses a wide variety of metal alloys, such as stainless steel, aluminum, and titanium. Some of these materials are more expensive than others.
- Investment casting is efficient with materials, often using lightweight alternatives that help lower overall production costs by reducing material usage.
Efficiency and Waste Reduction:
- The method is notable for its efficiency and minimal waste production. Unlike other manufacturing techniques, investment casting produces complex parts without requiring extensive machining or secondary operations, which further cuts down on material and labor costs.
Detailed and Precise Outputs:
- The wax patterns used in investment casting allow for intricate designs and fine details, which are exact replicas in the final metal parts. The ceramic molds maintain the geometry of the wax patterns, ensuring the cast parts retain their complex features.
- This precision is challenging to achieve with other manufacturing processes, making investment casting particularly valuable for applications requiring high detail.
How Does Investment Casting Stand Out from Other Casting Methods?
Investment casting is unique compared to other types of casting like die casting and sand casting. The key difference is how the mold is made. In investment casting, a wax pattern of the final part is created first. This wax model is then covered with a ceramic material that hardens into a solid mold. Die casting, on the other hand, involves forcing molten metal under high pressure into a metal mold material. Sand casting uses a mixture of sand and a binder packed around a pattern to shape the mold cavity.
The precision and detail in investment casting are higher than in die casting and sand casting because the wax model can capture intricate details. This method is especially good for making thin-walled parts without any holes or gaps, achieving high accuracy.
Investment casting combines the benefits of detailed accuracy and reasonable costs, making it ideal for complex shapes and parts that need tight tolerances. It also allows for using various metal alloys, giving manufacturers flexibility in choosing materials.
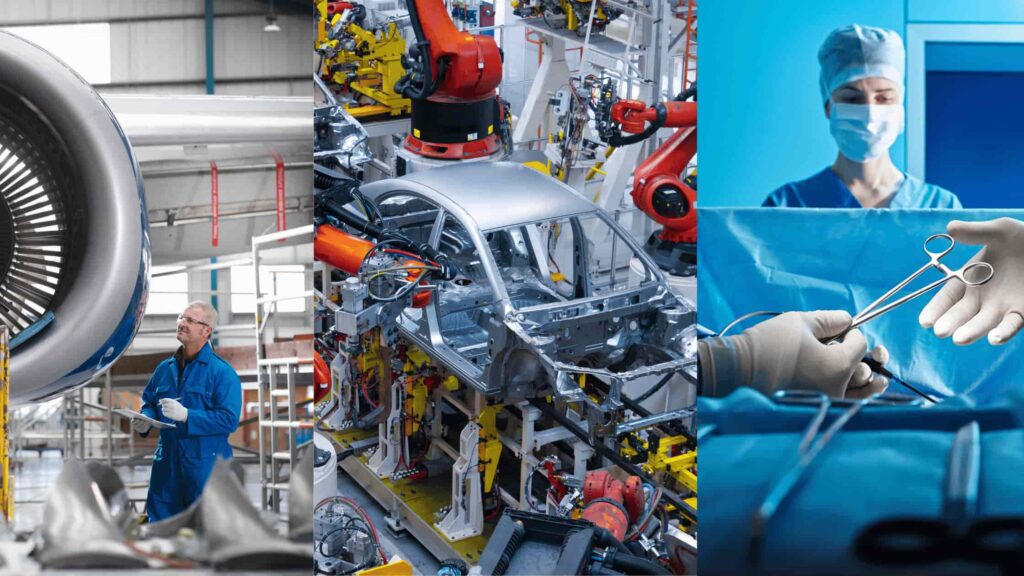
Which Industries Rely on Investment Casting?
Investment casting is popular across various sectors, including aerospace, automotive, and medical industries. In aerospace, it is used to make complex parts like turbine blades and engine components, offering excellent precision and minimal waste. Automotive manufacturers use investment casting for parts like cylinder heads and transmission components, benefiting from its ability to create lightweight and high-performance designs. The medical field also uses this technique for producing detailed implants and surgical tools, ensuring high precision and reliability essential for medical devices.
Exploring the Durability of Investment Casting Products
Precision casting products are renowned for their exceptional durability. This durability can be attributed to several factors including design, operational conditions, and material selection.
- Design Considerations: The durability of the products largely depends on their design. A well-thought-out design incorporates elements such as stress distribution, load-bearing capacity, and structural integrity. For instance, brass bearing surfaces in machinery are specifically designed to handle high loads and provide frictionless operation, which contributes to their longevity.
- Operational Limitations: The operating conditions that a product will face play a crucial role in determining its durability. Products are designed with these conditions in mind, ensuring they can withstand specific forces, vibrations, and environmental exposures. A prime example is automotive suspension knuckle joints, which are engineered to endure constant vibrations and harsh environmental conditions, thus enhancing their durability.
- Material Selection: Selecting appropriate materials is critical for the durability of investment casting products. The chosen materials must possess the necessary mechanical properties and corrosion resistance to ensure the product’s longevity. Stainless steel, for example, is often used in the process for its excellent strength, toughness, and resistance to corrosion.
- Additional Factors Contributing to Durability: It also allows for the creation of complex geometries, which can optimize the functionality and therefore the durability of the products. The process ensures precise dimensional accuracy, which is essential for the correct fit and alignment of components, enhancing both performance and durability. Furthermore, investment casting minimizes material wastage and facilitates the use of high-quality materials, both of which are instrumental in improving the structural integrity of the final product.
- Heat Resistance: The durability is also influenced by their ability to resist heat. This is dependent on the materials used; certain materials such as superalloys are chosen specifically for their exceptional heat resistance properties. These materials ensure that the products can operate effectively in high-temperature environments without degrading.
In summary, investment casting is a versatile process used in industrial applications for creating parts with different metals. It has size and cross-sectional limits, making it suitable for small to medium-sized parts. Correct product choice and strict process and quality control procedures are vital for successful investment casting.
Shakeout, Cleaning, and Finishing: Revealing the Cast
After the metal has cooled and solidified within the mold, the next phase is one of transformation, where the rough cast emerges from its sandy cocoon, ready to undergo the metamorphosis into a finished piece. This phase, comprising the shakeout, cleaning, and finishing processes, is crucial for revealing the cast’s true form and preparing it for its intended function. Let’s explore these steps that take the casting from a raw state to a polished final product.
The Shakeout Process: Separating the Cast Part from the Sand Mold
The shakeout is the first step in uncovering the newly formed metal part, a process that involves breaking away the sand mold to reveal the casting within. This step requires careful handling to ensure the integrity of the cast part is maintained. Mechanical vibrators, shakeout machines, or simple manual methods are employed to remove the sand, which can be reclaimed and reused for future molds. The objective is to efficiently separate the sand from the cast without causing damage to the delicate new creation.
Cleaning Operations: Trimming, Sandblasting, and Surface Treatments
Once freed from the majority of the sand, the cast part often requires further cleaning to remove any remaining sand particles, as well as the removal of excess metal such as gates, runners, and flash. This is where cleaning operations come into play:
- Trimming: Excess metal that formed in the mold’s seams or at the inlets and outlets of the mold is cut away, usually by sawing or grinding.
- Sandblasting: To remove any clinging sand and improve the surface texture, the casting is subjected to sandblasting. This process involves blasting the surface with a high-pressure stream of abrasive material, leaving behind a clean, textured surface.
- Surface Treatments: Additional treatments may be applied to enhance the casting’s surface properties, such as acid etching or shot peening, which can help to improve fatigue resistance.
Finishing Touches: Machining, Painting, or Coating for Aesthetic and Functional Purposes
The final step in the casting process is finishing, where the cast part is refined to meet the precise specifications required for its intended use. This can involve a variety of processes, tailored to the demands of the final product:
- Machining: Precision machining is often necessary to achieve the exact dimensions and tolerances needed for the casting to function as designed. Processes like milling, drilling, and turning are used to refine the shape and size of the part.
- Painting or Coating: For aesthetic enhancement or to provide a protective layer against corrosion, castings may be painted or coated with various materials. This can range from simple paint applications to complex chemical coatings designed to withstand extreme environments.
- Quality Inspection: Finally, the casting undergoes a thorough inspection to ensure it meets all quality standards and specifications. This may include visual inspection, dimensional measurement, and non-destructive testing methods.
Through the meticulous processes of shakeout, cleaning, and finishing, the casting is transformed from a rough, sand-encrusted object into a precise, polished component, ready to take its place in the world. This journey from mold to masterpiece underscores the blend of brute force and delicate precision that defines the art and science of sand casting.
Cooling and Solidification: A Delicate Transition
After the exhilarating moment of pouring molten metal into the mold, the sand casting process enters a phase of quiet transformation: cooling and solidification. This stage is pivotal, as the characteristics of the final casting are largely determined by the events that occur as the metal transitions from liquid to solid. Understanding the science of cooling curves and solidification patterns, alongside managing the factors that influence cooling rates, is essential in producing high-quality castings.
Explanation of Cooling Curves and Solidification Patterns
Cooling curves are graphical representations that show how the temperature of the metal changes over time as it cools and solidifies in the mold. Each metal or alloy has a unique cooling curve, influenced by its chemical composition, which dictates the rate at which it must be cooled to achieve desired properties. Solidification patterns, on the other hand, describe the manner in which the metal transforms from liquid to solid. These patterns can reveal insights into the potential for defects, the formation of different microstructures, and the overall quality of the cast.
Factors Affecting Cooling Rates and Their Impact on Casting Quality
Several factors influence how quickly and uniformly a casting cools, each affecting the casting’s final characteristics:
- Mold Material: The thermal conductivity of the mold material plays a significant role. Sand molds, with relatively low thermal conductivity, cool the metal slower than metal molds, affecting the microstructure and mechanical properties of the casting.
- Casting Thickness: Thicker sections of a casting cool more slowly than thinner sections, leading to variations in microstructure and potential for defects such as shrinkage cavities.
- Temperature of the Molten Metal: The initial temperature of the molten metal when poured can influence the cooling rate; higher temperatures result in longer cooling times.
- Environmental Conditions: Ambient temperature and airflow around the mold can also affect cooling rates.
These factors can lead to a range of casting defects if not properly managed, including porosity, inclusions, and uneven mechanical properties.
Strategies to Control Cooling and Avoid Defects
To mitigate the risk of defects and ensure a high-quality casting, several strategies can be employed to control cooling rates:
- Chills: These are materials with high thermal conductivity that are placed in the mold to accelerate cooling in specific areas of the casting. Chills can be used to create more uniform cooling rates across the casting, preventing areas of localized shrinkage.
- Insulation: Conversely, insulating materials may be used to slow the cooling rate in certain sections of the casting to avoid thermal stresses and cracking. This is often necessary for castings with varying cross-sectional thicknesses.
- Controlled Pouring Temperature: Adjusting the temperature of the molten metal to the optimal pouring temperature for the specific alloy and casting size can help manage the cooling rate.
- Mold Design Adjustments: Modifying the design of the mold, including the thickness and placement of the sand, can help achieve more uniform cooling.
Cooling and solidification are not merely passive stages in the sand casting process; they are phases ripe with potential for influencing the final outcome. Through a deep understanding of the underlying principles and a strategic approach to managing cooling rates, foundry workers and artisans can significantly enhance the quality and integrity of their castings. This delicate transition, when navigated with skill and knowledge, marks the birth of a new metal form, ready to be revealed to the world.
Pouring Metal: From Liquid to Solid
The transformation of molten metal into a solid form is both a critical and captivating phase in the sand casting process. This stage is where the preparatory work of pattern making and mold preparation converges with the alchemy of metalwork. Let’s explore the intricacies of pouring metal, focusing on preparation, techniques, and considerations crucial to achieving a defect-free casting.
Preparing for Pouring: Checking Metal Temperature and Mold Readiness
Before the molten metal is introduced into the mold, two pivotal checks must be performed: ensuring the metal is at the correct temperature and confirming the mold’s readiness.
- Metal Temperature: The temperature of the molten metal is crucial for a successful pour. If the metal is too hot, it may cause unwanted reactions with the mold material or result in defects like gas porosity. If too cold, the metal might solidify before filling the entire mold cavity. Using a pyrometer, the temperature is precisely measured to align with the specific metal’s ideal pouring range.
- Mold Readiness: The mold must be dry and free of contaminants. Moisture can cause steam explosions, while dirt or debris can lead to surface defects in the final casting. The mold’s integrity is inspected to ensure it can withstand the thermal shock of the molten metal without collapsing or cracking.
Techniques and Tools for Pouring: Ladles, Pouring Basins, Gates, and Runners
The actual pouring of the metal requires skill and the right tools to direct the molten metal from the furnace to the mold cavity efficiently.
- Ladles: These are the primary tools for transporting and pouring molten metal. They range from simple hand-held devices for small quantities to mechanically operated ladles for large volumes, ensuring precise control over the pour.
- Pouring Basins: A funnel-shaped reservoir at the top of the mold that receives the molten metal from the ladle. It helps in minimizing splashing and directs the flow into the mold’s gating system.
- Gates and Runners: These are channels carved into the sand mold that guide the molten metal from the pouring basin to the mold cavity. Proper design of gates and runners is vital to control the flow speed and reduce turbulence.
Considerations to Avoid Defects: Turbulence, Air Entrapment, and Temperature Control
The way metal is poured can significantly impact the quality of the casting. Here are key considerations to mitigate common defects:
- Turbulence: A gentle and controlled pour is essential to prevent the molten metal from splashing and creating turbulence as it enters the mold. Turbulence can lead to air entrapment and oxide formation, compromising the casting’s integrity.
- Air Entrapment: Strategic placement of vents in the mold allows gases to escape as the molten metal fills the cavity. Ensuring an uninterrupted flow of metal helps in minimizing air pockets or voids in the final casting.
- Temperature Control: Besides the initial temperature check, maintaining an optimal temperature gradient during the pour is crucial. Too rapid cooling can lead to cold shuts (where liquid metal streams don’t fuse properly), while too slow can cause shrinkage cavities as the metal solidifies.
Mastering the pour is a delicate balance between art and science, requiring attention to detail, precision, and timing. When executed correctly, it culminates in the magical moment where molten metal begins its transformation into a solid, embodying the desired shape and properties embedded in the sand mold. This phase is not just about transferring metal from one container to another; it’s about ensuring the integrity, quality, and success of the casting through meticulous preparation and execution.
Melting Metal: The Heart of Sand Casting
At the heart of sand casting lies the transformative process of melting metal, turning solid ingots or scraps into a fiery liquid form ready to take on a new identity. This stage is where raw materials are prepped to become intricate parts of machinery, beautiful sculptures, or essential everyday tools. Let’s explore the critical aspects of melting metal, from selecting the right type of metal to understanding the intricacies of the melting process and the final adjustments before the pour.
Overview of Metal Selection
The choice of metal is pivotal and depends on the desired properties of the final cast product. Each metal or alloy offers a unique combination of strength, ductility, corrosion resistance, and thermal conductivity. The most commonly used metals in sand casting include:
- Iron: Renowned for its strength and durability, iron is often used in automotive and machinery components.
- Aluminum: Known for its lightweight and corrosion resistance, aluminum is favored in aerospace and automotive industries.
- Bronze: Valued for its excellent corrosion resistance and aesthetic appeal, bronze is commonly used in sculptures and marine hardware.
The selection process involves considering the application’s requirements and balancing them with cost and material properties to choose the most suitable metal.
The Melting Process
Melting metal is a complex operation that demands precision and safety. The process varies depending on the metal, but the fundamentals remain the same. Here’s a breakdown:
- Furnaces Used: Different types of furnaces are employed based on the metal’s melting point and the volume of metal needed. Common furnaces include cupola furnaces for iron, electric arc furnaces for steel, and induction furnaces for a variety of metals. Each offers advantages in efficiency, temperature control, and environmental impact.
- Safety Measures: Melting metal involves extremely high temperatures, necessitating strict safety protocols to protect workers. Personal protective equipment (PPE), such as heat-resistant gloves, face shields, and aprons, are essential. Proper ventilation and training in handling molten metal are also critical to prevent accidents.
- Temperature Control: Precise temperature control is crucial for achieving the desired metal properties. Each metal and alloy has an optimal pouring temperature to ensure it fills the mold cavity correctly without premature solidification or creating defects.
Alloying and Treatment
The composition of the metal significantly influences the properties of the final casting. Adjustments can be made during the melting process to achieve the desired characteristics:
- Alloying: Adding specific elements to the base metal can enhance its strength, ductility, or corrosion resistance. For instance, adding chromium and nickel to steel improves its stainless properties, while adding silicon to aluminum can increase its casting ability.
- Treatment: Metal treatments, such as degassing or fluxing, are often performed to improve the quality of the melt. Degassing removes dissolved gases that could cause porosity in the casting, and fluxing cleans the metal by removing impurities to the surface, where they can be skimmed off.
The melting stage in sand casting is both a science and an art, requiring deep knowledge of materials and meticulous attention to detail. By carefully selecting the metal, mastering the melting process, and fine-tuning the composition, casters ensure the molten metal is perfectly primed for its transformation into a solid, new form within the sand mold. This pivotal phase not only shapes the metal’s destiny but also reflects the profound skill and understanding behind the ancient craft of sand casting.
Mold Preparation: Crafting the Cavity
After the meticulous creation of a pattern, the next step in the sand casting process is preparing the mold—a critical phase where the sand meets the design to create a negative space for the molten metal. This stage is all about precision and technique, ensuring that the final cast will be as close to the original design as possible. Let’s explore the intricacies of mold preparation, from the selection of sand to the creation of cores for internal cavities.
Introduction to Sand Types and Properties
The foundation of a good mold is the sand used to make it. The choice of sand significantly affects the mold’s quality, impacting the surface finish, dimensional accuracy, and strength of the final casting. Two of the most common types of sand used in casting are:
- Green Sand: A mixture of silica sand, clay (binder), and water, green sand is called so not because of its color but because it is ‘wet’ or ‘fresh’ when the mold is made. It’s reusable and suitable for a wide range of casting metals and sizes.
- Resin Sand: Enhanced with a resin binder instead of clay, resin sand provides higher strength and better dimensional accuracy. It’s often used for larger castings or when higher precision is required.
Each type of sand has its own set of properties—like permeability, cohesiveness, and thermal stability—that make it suitable for specific casting applications.
Mold Components: Cope and Drag
The sand mold consists of two primary parts: the cope (the top half) and the drag (the bottom half). These terms aren’t just jargon but signify the functionality of each part of the mold. The cope and drag work together to form the entire mold cavity, where the drag supports the weight and the cope contains the risers and gating system necessary for the metal to enter and gases to escape.
The Process of Packing Sand Around the Pattern: Ramming and Compaction
Creating a mold starts with placing the pattern in a mold box (flask) and then packing it with sand. The key to this step is ensuring the sand is compacted tightly around the pattern to capture every detail:
- Filling: The flask is filled with sand around the pattern. Special attention is paid to ensure the sand gets into all the crevices and details of the pattern.
- Ramming: The sand is compacted, or “rammed,” around the pattern, usually with a pneumatic rammer, to ensure it’s firm and there are no gaps.
- Removal: Once the sand has been sufficiently compacted, the pattern is carefully removed, leaving a cavity in the shape of the part to be cast.
This process might be repeated for both the cope and drag sections of the mold, depending on the complexity of the casting.
Core Making: Materials, Making, and Placement for Internal Cavities
For castings that require internal cavities or complex geometries, cores are used. Cores are made from similar materials as the mold but are often strengthened with binders or other additives to maintain their shape under the pressure of molten metal:
- Materials: Core sand is selected based on the casting material and the required surface finish and strength of the core.
- Making: Cores are formed using a core box, similar to the pattern in mold making. The sand is packed into the core box and then hardened, usually with heat or chemical binders.
- Placement: Once hardened, the core is placed into the mold cavity before the mold is closed. It’s positioned so that, once the metal is poured, it will fill around the core, creating the desired internal structure.
Mold preparation is a testament to the craftsmanship and technical skill involved in sand casting. Every step, from selecting the right type of sand to precisely packing it around the pattern and carefully placing cores, requires a deep understanding of the materials and processes involved. This phase sets the stage for the magic of metal casting, where the visions of designers and engineers come to life in solid form.